Genotype–phenotype Correlations and Treatment Innovation of Neurofibromatosis Type 1 (NF1): Current Understanding and Perspective
Chinese Medical Journal(2024)
摘要
Neurofibromatosis type 1 (NF1) is an autosomal dominant inherited disease caused by mutations in the NF1 gene. It is characterized by cafe-au-lait macules (CALMs), cutaneous neurofibromas (CNF), plexiform neurofibromas (PNF), Lisch nodules, optic pathway gliomas (OPG), potential skeletal abnormalities, and other complications involving multiple organs and systems.[1] Additionally, approximately 10% of individuals with NF1 may progress to develop malignant peripheral nerve sheath tumors (MPNST).[1] The NF1 gene encodes neurofibromin, which regulates Ras and its downstream signaling pathways, such as the mitogen-activated protein kinase (MEK)/extracellular signal-regulated kinase (ERK) and phosphatidylinositol-3-kinase (PI3K)/Akt/mammalian target of rapamycin (mTOR) pathways, thereby inhibiting abnormal cell differentiation and proliferation.[2,3] Unfortunately, because of the high clinical and genetic heterogeneity of the disease, the genotype–phenotype correlation in NF1 patients remains elusive, posing challenges for diagnosis, treatment, and genetic counseling. With the growing popularity of genetic testing and comprehensive investigations into molecular mechanisms, more genotype–phenotype correlations have been elucidated, and breakthroughs have been made in targeted therapeutics for NF1-related PNF. Current understanding of genotype–phenotype correlations: A limited number of established genotype–phenotype correlations in NF1 were revealed. The first identified genotype–phenotype correlation was NF1 microdeletion syndrome, which involves the deletion of large segments of the NF1 gene and its flanking region.[4] This syndrome is estimated to affect 5–11% of NF1 patients and is associated with more severe phenotypes including a large number of CNF, a greater burden and earlier onset of PNF and spinal neurofibromas, and an increased risk and earlier onset of MPNST and other malignancies.[4,5] Missense mutations at codons 844–848 also lead to severe phenotypes, marked by an increased prevalence of PNF, symptomatic spinal neurofibromas, OPG, MPNST, and skeletal abnormalities.[6] Specifically, missense mutations at p.Lys1423 are associated with an increased likelihood of major external PNF and pulmonary artery stenosis, while p.R1241* is correlated with structural brain alterations.[5,7] The p.Y2285* mutation is linked to a greater risk of Lisch nodules and endocrinological disorders, and individuals with the p.R1748* mutation exhibit a higher probability of developing PNF and MPNST.[5,8] Patients with splice site mutations exhibit a greater risk of spinal neurofibromas, brain tumors, and connective tissue tumors.[8] Moreover, mutations involving the protein kinase C (PKC) domain of neurofibromin predispose patients to CNF, Lisch nodules, PNF, and psychiatric disorders, and involvement of the cysteine-serine rich domain (CSRD) domain may increase the risk of OPG and secondary primary malignancies.[8] The involvement of the GTPase-activating protein-related domain (GRD) is significantly associated with an increased occurrence of Lisch nodules, and the involvement of the Sec14-pleckstrin homology (PH) domain is correlated with a greater incidence of CNF.[8] Conversely, certain genetic mutations are associated with milder phenotypes. For instance, patients with the p.Met992del mutation and missense mutations at p.Met1149 and p.Arg1809 exhibit no CNF or obvious PNF, presenting only pigmentary manifestations, with the latter two mutations also showing Noonan-like features and short stature.[7,9] Missense mutations at p.Arg1276 are associated with a greater prevalence of the Noonan-like phenotype and a lower incidence of CNF.[7] Missense mutations generally lead to fewer Lisch nodules, CNF, and PNF than other mutation types.[5,10] Development of targeted therapies for NF1-PNF: Patients suffer greatly because a large percentage of PNF cannot be operated on, due to the rich blood supply, locally invasive growth characteristics of PNF, and the tendency to grow along the trunk of large nerves, which compresses the surrounding tissues and organs. With recent advancements in the understanding of the molecular mechanisms and genetic factors of this disease, targeted therapy for NF1-related PNF has become possible, bringing hope to PNF patients with large tumors, functional impairment, and compression symptoms. The potential therapeutic targets of MEK have been explored in multiple clinical studies and trials for NF1-related PNF. Among these targeted therapies, the highly selective MEK1/2 inhibitor selumetinib has emerged as a particularly notable agent. In phase I clinical trials, 71% (17/24) of patients experienced a partial response (PR), which means that the volume of PNF decreased by at least 20%.[11] In phase II, 74% (37/50) of the participants achieved PR, and the overall response rate reached 68%, indicating that there was at least one improvement in the patients' overall changes, motor function, quality of life, and pain.[12] Moreover, its adverse reactions are mild and acceptable. These exciting results prompted the Food and Drug Administration (FDA) and the National Medical Products Administration (NMPA) to approve selumetinib in May 2020 and April 2023, respectively. This is also the first and only NF1 medication available for treating pediatric NF1 patients aged two years and older with symptomatic and inoperable PNF. Furthermore, additional MEK inhibitors are under investigation. Trametinib demonstrated encouraging results in pediatric patients, with a 46% (12/26) PR rate.[13] Mirdametinib and FCN-159 have shown effectiveness in patients older than 16 years and 18 years with PRs reaching 42% and 37.5%, respectively, and both are capable of reducing pain.[14,15] This could be a breakthrough for the NF1 adult population. There are also other active clinical trials being conducted such as binimetinib. More clinical trials are currently being conducted to verify its efficacy and safety, and efforts are being made to expand its indications [Supplementary Table 1, https://links.lww.com/CM9/C66]. Simultaneously, various targeted drugs, which target other molecules, have been examined [Supplementary Table 2, https://links.lww.com/CM9/C66]. Regrettably, other medications have not yet demonstrated adequate efficacy, with the exception of cabozantinib, which improved on the general pain and hurt scale and demonstrated a PR of 42%.[16] MEK inhibitors, such as selumetinib, have achieved breakthrough in the treatment of NF1-related PNF, positioning pharmacotherapy as a viable alternative to surgical intervention in clinical decision-making and management. The identification of genotype–phenotype correlations within NF1 clinical cohorts could revolutionize the diagnosis, personalized treatment, genetic counseling, and prognosis of NF1 patients. This advancement not only enhances our understanding of NF1 gene functions and the pathophysiology of the disease but also heralds a new era of genetic-driven precision medicine in NF1 treatment. Advancement of gene therapies for NF1-PNF: Given the significant heterogeneity of NF1, gene therapy has emerged as a particularly promising treatment for this complex monogenic disorder. Gene therapy aims to halt tumor growth and development by restoring the activity and function of the neurofibromin protein through the replacement or repairment of gene sequences. This approach reduces the likelihood of neurofibroma malignancy and recurrence, alleviating the symptoms and increasing the functionality of NF1 patients. It's critical to conduct gene therapy during the embryonic and early infant stages, offering the potential for long-term or even lifelong absence of tumors if the pathogenic gene is corrected. However, the clinical reality often involves managing existing PNF, where gene therapy can still effectively control symptoms and inhibit further growth. Gene therapy encompasses transgenic and gene-editing techniques. Transgenic therapy involves integrating exogenous functional genes into cells with genetic defects using vectors such as adeno-associated viruses, and gene editing employs precision technologies such as clustered regularly interspaced short palindromic repeat (CRISPR)/CRISPR-associated protein 9 (Cas9) for targeted modification. Although gene therapy is still in an exploratory stage and its efficacy and safety require further validation, its potential and prospects are immense. Continuous technological innovation will develop more efficient transgenic delivery systems and precise gene-editing tools, benefiting NF1 patients while maintaining a focus on both technology and ethics. Many unanswered questions remain to be explored in the NF1 field, including understanding the natural history of NF1, defining comprehensive phenotypes, and quantifying disease burden. Although existing genotype–phenotype correlations and trials of targeted medicine are supported by clinical data, large-scale, prospective, multicenter studies are needed for further investigation and validation. Additionally, it is necessary to emphasize data integration, emphasizing comprehensive clinical examinations, uniform genetic variation codes, and standardized phenotype descriptions in the collaborations. Besides, the necessity for continued functional analyses and revealing the underlying mechanisms cannot be overstated. The impact of variants on functional domains and downstream signaling pathways is yet to be fully elucidated, marking a vital area for future research in the quest to fully understand and combat NF1. In summary, NF1 shows a high degree of clinical diversity and genetic heterogeneity. Existing genotype–phenotype correlation studies have enhanced our understanding of the multifaceted impacts of the variant. Further research is imperative to guide the development of more effective and safer targeted and gene therapies, thereby improving screening, counseling, monitoring, and intervention strategies for NF1 patients. Funding This work was supported by grants from the National Natural Science Foundation of China (Nos. 82102344 and 82172228), Shanghai Clinical Research Center of Plastic and Reconstructive Surgery supported by the Science and Technology Commission of Shanghai Municipality (No. 22MC1940300), Innovative research team of high-level local universities in Shanghai (No. SHSMU-ZDCX20210400), Natural Science Foundation of Shanghai (No. 22ZR1422300); Shanghai Municipal Key Clinical Specialty (No. shslczdzk00901), and the Project of Biobank (No. YBKA202204) from Shanghai Ninth People's Hospital, Shanghai Jiao Tong University School of Medicine. Conflicts of interest None.
更多查看译文
AI 理解论文
溯源树
样例
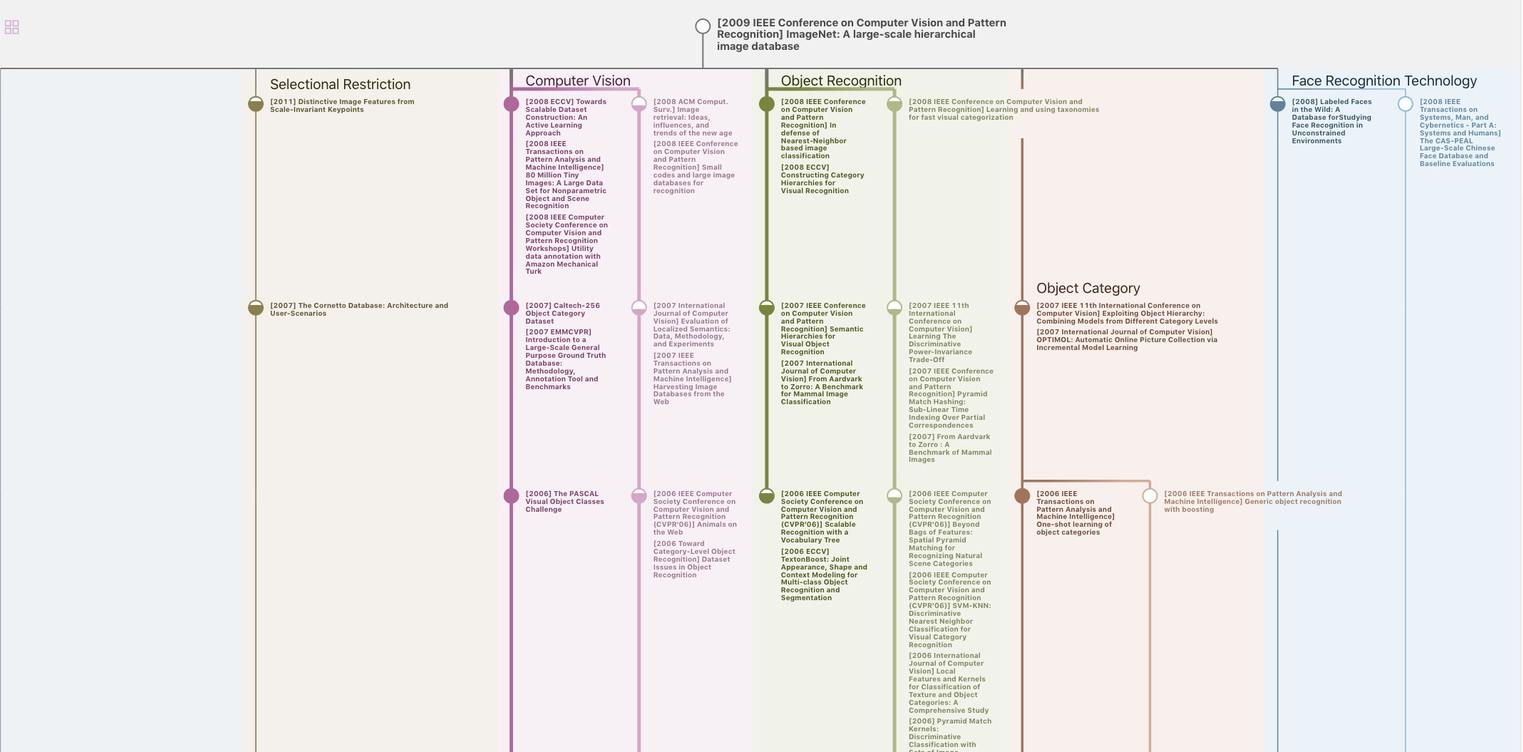
生成溯源树,研究论文发展脉络
数据免责声明
页面数据均来自互联网公开来源、合作出版商和通过AI技术自动分析结果,我们不对页面数据的有效性、准确性、正确性、可靠性、完整性和及时性做出任何承诺和保证。若有疑问,可以通过电子邮件方式联系我们:report@aminer.cn